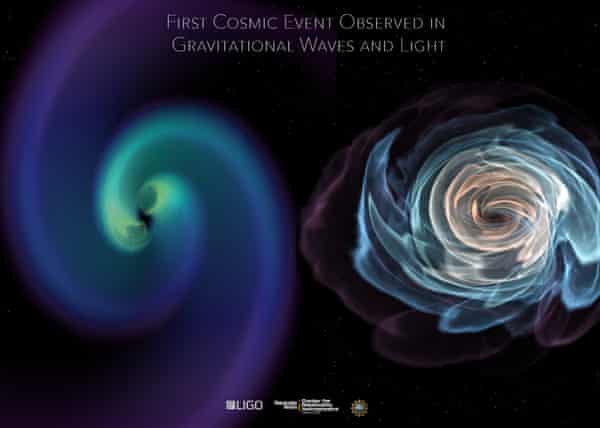

Diagram of laser interferometer such as employed by LIGO (from Wikipedia)
The news that a collision of two neutron stars has precipitated another detection of gravitational waves by LIGO (Laser Interferometer Gravitational Wave Observatory), has the world of astrophysics abuzz and with good reason. It confirms the import of the laser interferometer principle used in gravitational wave detection (see diagram 2) and biw in the setting of another type of collision- between two neutron stars. Recall the original report occurred in February, last year, based on detecting gravitational waves from two colliding black holes in late 2015.
LIGO’s original discovery, accepted for publication in Physical Review Letters,
Based on the data cited in the above link, the two black holes were each roughly 30 times the mass of the Sun. They evidently merged some 1.3 billion light years from Earth. The gravitational waves themselves were generated in the final moments before the black holes merged. The signal was brief but definitive and we on Earth have now received it.
LIGO features 2 L-shaped detectors (one in Hanford, WA, the other in Livingston, LA) made up of two perpendicular arms totaling 2.5 miles long. Then a laser beam is split and travels along both arms, bouncing off respective mirrors to return to the L-intersection. Normally, the beams are aligned so they balance each other out and hence there's nothing to detect. But if a gravitational wave is intercepted it creates a tiny mismatch which is what LIGO detects. (One of the authors of the paper has referred to it as a "chirp". The effect of this chirp or ripple changes the arms' lengths by a tiny amount, and that change can be detected by lasers.)
To determine the specific location of the source multiple detectors are used, both to distinguish signals from other "noise" by confirming the signal is not of earthly origin, and also to determine direction by means of triangulation. In such triangulations a third LIGO site is used, namely one near Pisa, Italy, with a 3 km interferometer. The technique uses the fact that the gravitational waves travel at the speed of light and will reach different detectors at different times depending on their source direction. Although the differences in arrival time may be just a few milliseconds, this is sufficient to identify the direction of the origin of the wave with considerable precision.
In 1915 Albert Einstein, in a remarkable achievement of theoretical physics, used an abstruse form of math known as tensor calculus to predict the existence of gravitational waves. This was by way of showing how gravitational distortions arise when mass or energy warp space-time. The ground breaking field equation that relates these parameters was summarized:
G mn = - ½ g mn G= - 8 p T mn
Where the T mn denotes the associated “stress-energy” tensor which incorporates internal stresses, the density of matter and its component velocities (u, v, w or in some texts: u1, u2 and u3).
Now, the collision of a pair of neutron stars has again brought gravitational waves front and center given it may finally resolve how the heaviest elements originated. The collision itself occurred some 130 million years ago when dinosaurs still walked the Earth, but the signal was only detected on August 17, 2016 given light propagates at a finite speed: 300,000 km/s.
Recall that the current theory of stellar evolution postulates that elements are built up in the cores of heavy stars (> 10 solar masses)by nucleosynthesis a la successive fusion reactions. A key transition point occurs after carbon is formed in the core, and reaches a critical density and temperature to detonate. The resulting deflagration, which includes the core separating from the exploding outer layers, turns the star into an instant nuclear factory. Nickel and iron are formed as well as lighter elements in the imploding shells including of: magnesium, sulphur, silicon, manganese, chromium and a host of lesser atomic weight elements- are evolved.
The problem is that no heavier elements than iron can be envisaged although some theoreticians have postulated they can arise in the context of supernova explositions. In the latter case a stage is reached whereby differing neutron fluences arise. These fluences, if conditions are right, can then produce the elements known as actinides such as uranium and thorium but no longer containing the A=130 abundance peak. This is believed to occur over seconds time scales via the r-(rapid neutron capture) process. But the only modern nearby supernova, 1987A, has not revealed r-process enhancements. Modern thinking is that the r-process yield may be ejected from some supernovae but swallowed up in others as part of the residual neutron star.
Note that the neutron star is an ultradense stellar remnant of a core-collapsed by supernova. The neutron star is the smallest, densest known to exist with diameter scales typically about 12 miles wide. One teaspoon of neutron star material would have a mass of about a billion tons. The core is a soup of pure neutrons, while the crust is smooth, solid and 10 billion times stronger than steel. Basically to achieve neutron star status protons and electrons are forced so closely together that they fuse, merge. e- + p + -> n.
So the current excitement is that the gravitational wave data from these colliding neutron stars may finally solve the heavier- than- iron elements origin problem. Dave Reitze, executive director of LIGO, said in an interview with the Guardian: “What is amazing about this discovery is it is the first time we’ve got a full picture of one of the most violent, cataclysmic events in the universe. This is the most intense observational campaign there has ever been.”
The 100-second hum picked up by LIGO has told the story of how the two neutron stars to be, each slightly heavier than the Sun, approached their demise. Initially separated by 200 miles, they circled each other 30 times a second. As they whirled inwards, accelerating to 2,000 orbits each second, the signal rose in pitch like a train whistle or ambulance alarm approaching. Two seconds later, NASA’s Fermi space telescope picked up an intense burst of gamma rays, emitted as shockwaves propagating through jets of matter funneled out of the poles during the energetic impact of the collision.
The chief problem here is the estimate of the source volume. This is facilitated in the neutron star case given we have that the components are separated by less than 300 km and accelerating to 2,000 orbits each second. This necessitates a tiny volume and implies that a lot of mass-energy will be distorting the associated region of space-time, and in this case leading ultimately to a black hole from the collision. This distortion itself is bound up with the stress-energy tensor T mn.
According to Prof Andreas Freise, a LIGO project scientist at the University of Birmingham:
“Neutron stars are at this sweet spot between a star and a black hole. When two of them collide, we expect them to immediately collapse into a black hole, leaving behind a bit of dust and stuff.”
David Shoemaker, spokesman for the LIGO Scientific Collaboration, said: “It’s [probably] the first observation of a black hole being created where there was none before, which is pretty darn cool.”
The recent observations also herald a new era of rapid-response astronomy, in which transient and unexpected cosmic events can be observed in detail for the first time. When LIGO's software picked up a signal at 13:41 GMT on 17 August, Shoemaker was one of a small team at LIGO to be alerted by a ringtone on his phone reserved for when black holes or neutron stars collide.
Within an hour, the detection had been confirmed by Virgo, a European gravitational wave detector near Pisa, the source of the signal had been triangulated to a small patch of sky and a global alert was triggered.
Prof Stephen Smartt, of Queen’s University Belfast, had been leading a five-day observation run of supernovae on the New Technology Telescope at La Silla, Chile, when the news came in. Smartt’s team, and those on other telescopes, observed the faint new blob and measured its spectrum to assess the chemical composition. The blob was a fireball of radioactive heavy chemical elements, known as a kilonova, that had been blown out from the collision at one fifth of the speed of light shortly after the gamma ray burst.
As I pointed out earlier, previously astrophysicists had speculated that the sheer rapidity of neutron capture (via r-process) would be enough to force extra neutrons into the nuclei of atoms, forging heavy elements like uranium. . Later the conjecture altered to considering the sheer mega-force of neutron collisions in violent events to forge heavy elements like gold and platinum But until now this idea was purely theoretical. After all, what kind of violent cosmic event would be needed to make it work? In the words of Prof. Freise:
“People have been looking for that forever,”
Adding:
“This is the first real confirmation that heavy elements such as gold, platinum and uranium are either solely or predominantly produced in binary neutron star collisions. The wedding band on your finger or the gold watch you’re wearing was most likely produced a billion years ago by two neutron stars colliding. That’s pretty cool.”
Let's also reference that earlier this month, three U.S. astrophysicists (Rainer, Weiss, Barry C. Barish and Kip S. Thorne) who played a crucial role in the development of LIGO were awarded the Nobel prize in physics for the first detection of gravitational waves. Shoemaker pointed out that two of the new laureates – and others – had been working on the project long before it captured the world’s attention. As he observed:
“This kind of thing doesn’t happen because there are suddenly neat instruments. It’s decades of work and people working together in a collaborative way. It’s quite phenomenal.”
Indeed it is. For those interested the findings were published on Monday in a series of papers in journals including Science, Nature and Physics Review Letters. For an abstract of the work appearing in the latter, see:
https://journals.aps.org/prl/abstract/10.1103/PhysRevLett.119.161101
No comments:
Post a Comment